The wind is one of the most important factors in soaring. It determines whether the ridges will work, if wave might develop, whether convergence lines can be expected, how thermals will drift, if cloud streets may form, etc. If we misjudge the wind we may find ourselves in massive sink where we had expected a strong climb. Even in flat terrain, one cannot plan a cross-country flight without understanding the wind. In the mountains, the consequences could be much more dramatic. So we should remind ourselves of the basics: why is there wind anyway?
- Why is there wind anyway?
All wind is caused by pressure differences, which themselves originate from differences in air temperature. The air always tries to eliminate pressure differences, which causes a wind from high pressure areas towards low pressure areas.
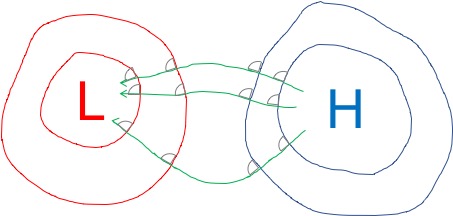
It is helpful to distinguish between winds occurring at different scales.
- What causes the wind on a global scale?
Pressure differences on a global scale originate from a) the differential heating between the equator and the poles, and b) the difference between the rotation speed of our planet’s surface and the rotational speed of the atmosphere, which is linked to it (Coriolis Effect).
If the Pressure Gradient Force were the only force in play, there would always be a straight wind from high pressure areas towards low pressure areas (see above). However, if we observe the winds on our rotating planet this is not the case because of the Coriolis Force. The effect of the Coriolis Force is that the trajectory of all objects (including air particles) moving through the atmosphere is deflected. In the Northern Hemisphere the deflection is to the right. In the Southern Hemisphere the deflection is to the left. It is strongest at the poles and reduces to nil at the equator. There is an animated graph on this Wikipedia page that is helpful to visualize how and why this occurs.
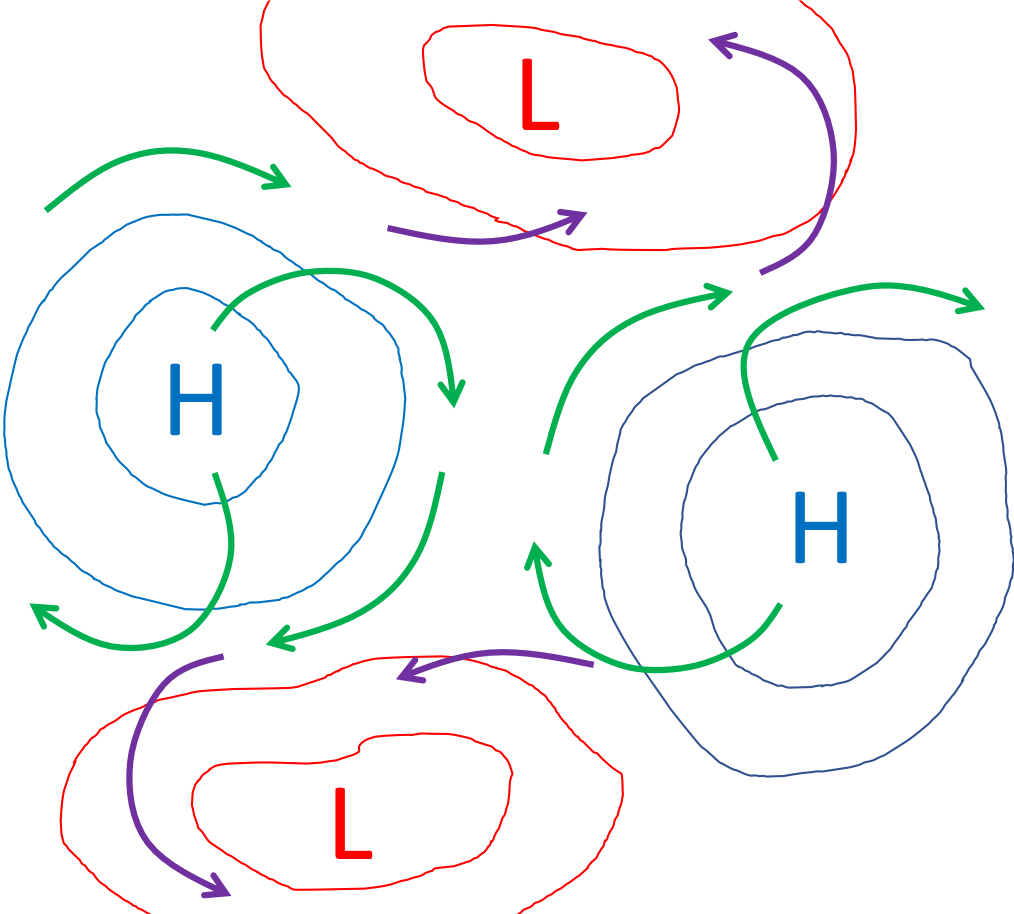
The effect of the Coriolis Force is that air flowing from high pressure areas towards low pressure areas is deflected: to the right in the Northern Hemisphere, and to the left in the Southern Hemisphere. The effect is that at an altitude of about 3,000 feet above the terrain (where there is little friction from the ground) the air will flow parallel to the isobars. In the Northern Hemisphere (as shown in the graph) that is clockwise around high pressure areas, and counterclockwise around low pressure areas. (These directions are reverse in the Southern Hemisphere).
At low altitudes the wind is subject to friction from the ground. The extent of the friction depends on the type of terrain (e.g. wooded hills vs flat desserts). This means that closer to the ground the wind speed will be reduced.
Because of the lower wind speed close to the ground the effect of the Coriolis Force is also gradually reduced. That means that the directional deflection will be less than at altitude. Close to the ground, the deflection of the wind is typically about 30 degrees less than at altitude. (The difference can range from 10 degrees over flat and smooth surface areas such as calm seas to about 40 degrees in mountainous terrain.)
In practical terms this means that we should not only expect the wind speed but also its direction to change between the ground and about 3,000 feet above: If the wind on the ground is blowing at 10 kt from 270 degrees, we might expect a wind speed of about 20kt at 3,000 feet with the wind now coming from approx. 300 degrees (in the Northern Hemisphere). This is, of course, a very general approximation and subject to many local factors (e.g. wind deflections by terrain features or weather factors such as convection, a potential ground inversion, etc.)
From a thermal soaring perspective, one is generally better off to be on the side of the low pressure areas where the air is “pulled in” (look at the purple arrows in the chart above), and not on the side of the high pressure areas where the air is “pushed out” (see the green arrows). On the low pressure side, i.e., where the air is “pulled in”, the air mass is rising (the air flow is cyclonic) and may produce better thermals. On the high pressure side, i.e. where the air is “pushed out”, the air mass is sinking (the airflow is katabatic) and the temperature gradient becomes stable such that thermal conditions deteriorate.
- What causes local and regional winds?
Since all wind is caused by pressure differences we need to ask what is causing such pressure differences at a more local or regional level.
The underlying reason are once again temperature differences due to differential solar heating. The following are some typical scenarios:
1. The Valley Breeze
The European Alps, the Colorado Rocky Mountains, as well as many other mountainous areas around the globe are characterized by bare mountains rising above vegetated hills, valleys, and the surrounding plains. In the mornings, sunshine begins to warm the sun-exposed flanks of the mountains well before it reaches down into the valleys where ground inversions tend to form overnight. Thermals begin to break-off from the mountains long before they can develop in the valleys. As columns of air begin to rise over the ridges and mountaintops, a “thermal low” is created that pulls in air from below to replace the air that is rising above the peaks.
In the summer, usually by mid-morning, air starts to flow from the valleys towards the mountains and begins to pull in more air from the surrounding plains. The air flowing upwards along the valleys is an anabatic wind called the Valley Breeze. In the Alps it can reach speeds of 10-15 kt on the ground, sometimes peaking around 20 kt in the afternoon and the biggest valleys. (I remember that at the airfield in Niederöblarn, Austria it is usually around 11:00 AM local daylight savings time in the summer months that all of a sudden the wind-sock comes alive as anabatic winds begin to move up along the valley. At this time, everyone rushes to their gliders to get ready for launch.)
Note that the air getting pulled in from the plains is typically warm and stable. That means it will always follow the path of least resistance, flowing around obstacles as much as possible rather than over them. The speed of the Valley Breeze also becomes slower the higher it gets pulled and the more it gets distributed into the secondary valleys further up in the mountains.
Ridge soaring in Valley Breeze alone is therefore mostly confined to relatively low altitudes, well below the peaks of the mountains.
Note that this flow system reverses at nightfall. The mountains cool off quickly and air starts to stream down along the slopes into the valleys (katabatic winds). Often, winds flowing down from the mountains on either side of the valley converge in the middle, causing air along the center of the valley to rise.
2. Sea Breezes
After sunrise, land masses heat up much more quickly than the sea because water absorbs solar energy only slowly. As the ground heats the air over the land, thermals develop and break off. The rising air creates a “thermal low” that needs to be filled by pulling in colder air from above the sea. The resulting anabatic wind is called Sea Breeze. Similar to the Valley Breeze, it usually reaches its maximum strength during the afternoon.
Where the sea breeze meets the dryer air mass further inland, convergence lines develop. This will be covered in the section about convergence lift.
Again, the system reverses at night. The land area cools off faster and a katabatic wind flow from the land towards the sea.
3. Monsoon Winds
While Valley Breezes and Sea Breezes are subject to a daily cycle caused by the rising and setting sun, Monsoon Winds are seasonal in duration and last several months. But otherwise there are the same principles at work.
E.g., Colorado is typically affected by the North American Monsoon, which causes an increase in rainfall and thunderstorm activity between the months of July and mid September. The monsoon season is caused by intense solar heating over the Mexican Plateau and the Southwestern United States causing a Thermal Low. The low pressure pulls in moist air from the Golf of California, and a strong High Pressure center over the Central United States pushes easterly winds and humidity from the Golf of Mexico towards New Mexico and Colorado.
The easterly flow is rarely strong enough to be used for ridge soaring along the eastern slope of the Colorado Front range. However, the meeting point between the humid easterly winds and the dryer air masses over the mountains often causes a convergence line that can be exploited for soaring purposes. This will be covered in the section about convergence lift.